How Mechanical Engineers Win a Pinewood Derby
Challenge Accepted:
Build the fastest pinewood derby car in the outlaw class – where the rules are looser, but the competition is fierce.
If you’re unfamiliar with Pinewood Derby, the races are governed by a simple set of rules: constraints on the car’s size, weight, and wheel configuration. For Stock class cars, the body must be constructed using the kit provided (block of wood, plastic wheels, nails for axles). In Outlaw class, the car can be constructed out of any material, which allows for some potentially more exciting cars. But in both classes, there can be no batteries, springs, motors, or anything other than gravity to propel the car.

Physics 101
The way this works, in a nutshell, is called Conservation of Energy. The car’s potential energy at the top of the hill is converted into kinetic energy on its way down to the bottom. Energy cannot be created or destroyed, only converted from one form into another. So, the simple way to explain this is:
Potential Energy (at top) = Kinetic Energy (at bottom)

The Equation
- m = mass of the car
- g = gravitational acceleration (~9.81m/s2)
- βh = change in height from the top to the bottom of the ramp
- v = velocity of the car at the bottom of the ramp
- In this case, mass (m) is unchanging, so it cancels out and you’re left with:
Velocity increases with the square root of the change in height. If the mass of your car starts higher and then ends low (like the other cars) you will have increased your change in height (ββ), which means your car will have a higher velocity (π£) at the bottom of the hill. Reviewing the rules, Mechanical Engineer Peter Nikiforov noticed there was nothing specified about the height of the car, and so he pitched an idea to his team: "what if the mass of our car started high and then ended low so that our ββ or potential energy was higher than everyone else’s?" More potential energy means more kinetic energy and therefore higher velocity at the bottom of the hill.

Whole lotta shakin' going on.
The group was initially skeptical of the proposed concept of a raised arm that would lower as the car rode down the track. Could an edge actually be gained by playing with height? Would that added complexity translate to better performance? Peter ran a series of simulations using a straightforward 2D dynamics analysis software that showed it would go faster than without the arm, but it had a major problem: the falling arm also made the car bounce and shake. That shaking means energy loss by vibration, friction, and to a lesser degree even sound.

So now there was a new challenge with only one week until the race. How to transfer the energy efficiently from our raised pendulum to the wheels without bouncing. The solution? A speed multiplying gear train.

“The pendulum only falls about 20 degrees, but we wanted to translate that motion into multiple full revolutions of the rear wheels so that even if the car was on a level surface it would still go forward.” That’s the power of gearing – and a little late-night engineering hustle. “I was up ‘til 2am on a work night dialing in the design. Then I printed it in the morning, assembled and tested it at my desk, and it worked really well.” Not only did it work well in testing, but it consistently won with an average speed of 207.45mph.
Solution delivered.
But the win wasn’t just in the results – it was the process. “It was much more about finding a creative engineering solution than just making the car perfect.” This shows what can happen when pairing classic physics with Sigma Design’s problem solving attitude. A unique solution to a well-tread challenge.
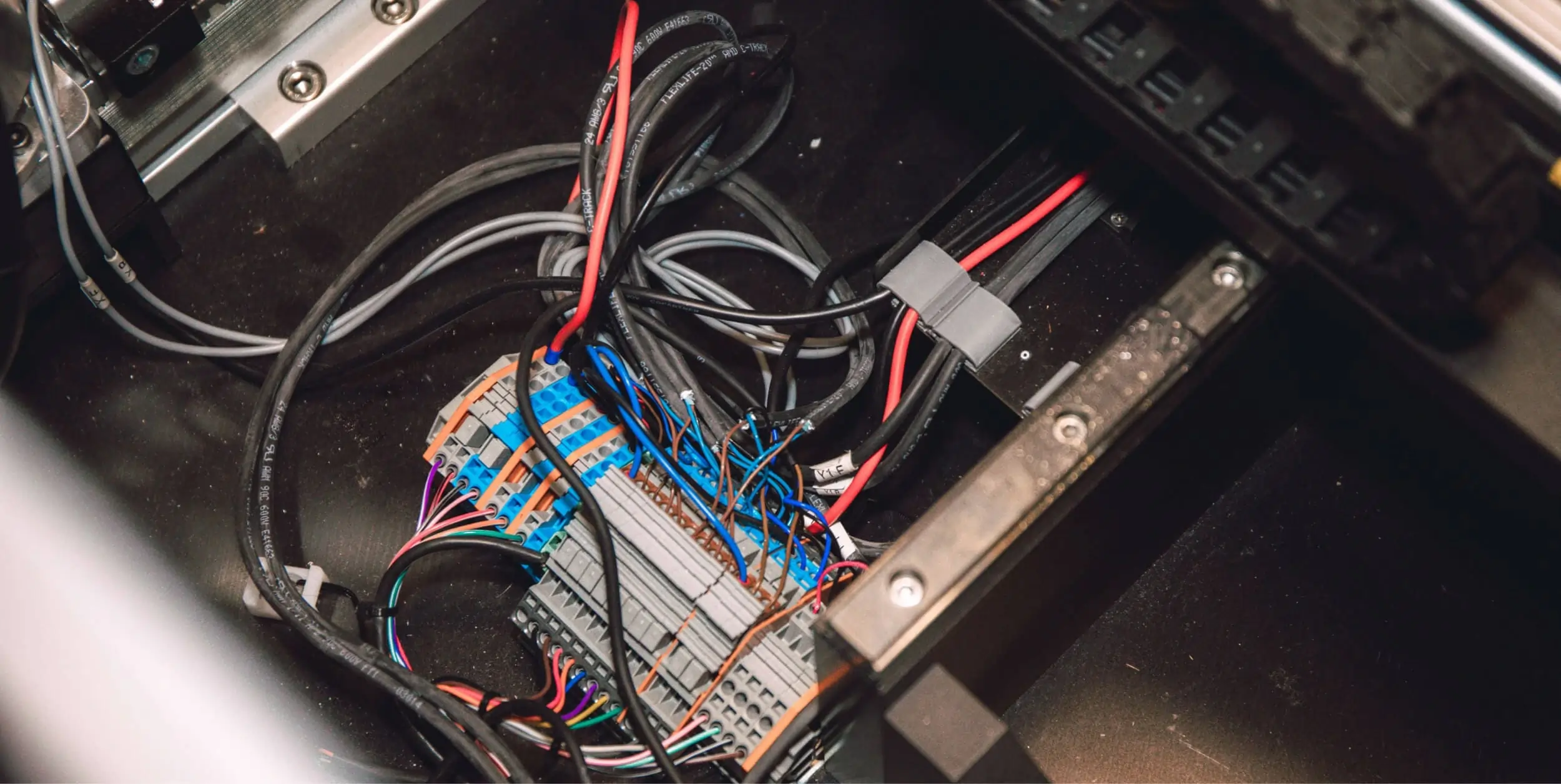